Robust Gigahertz Repetition Rate Optical Frequency Combs
Robust Gigahertz Repetition Rate Optical Frequency Combs
1. Introduction
High repetition rate (>1 GHz) optical frequency combs offer benefits over their lower repetition rate counter
parts in terms of faster data acquisition for remote sensing applications such as stand-off detection of hazardous compounds, geographic surveying, and optical ranging [1-3]. In addition, applications such as time and frequency transfer and ultra-low phase noise generation can also benefit from the higher pulse repetition rate. The larger separation of the comb teeth inherent to higher repetition rates combined with the frequency stability of the comb also supports the calibration of astronomical spectrographs towards the
search for earth-like exoplanets [4]. While GHz-level telecom-grade mode-locked oscillators are commercially available [5], the required optical pulse energy necessary to turn these lasers into fully stabilized optical frequency combs has been a significant hindrance to advancing this technology. The traditional detection of the carrier-envelope offset frequency (fceo) via supercontinuum generation in highly nonlinear fibers typically requires >600 pJ of pulse energy which, for a 1 GHz pulse train, is barely achievable with core-pumped fiber amplifiers (requiring > 600 mW of average power). While cladding-pumped amplifiers can achieve these power levels, they can easily be damaged and require free-space dispersion management to achieve <100 fs pulse durations. The pulse energy requirement adds size, complexity, and cost to these systems making them prohibitive to field deployment due to environmental sensitivities as well as being too costly to fit within many experimental budgets.
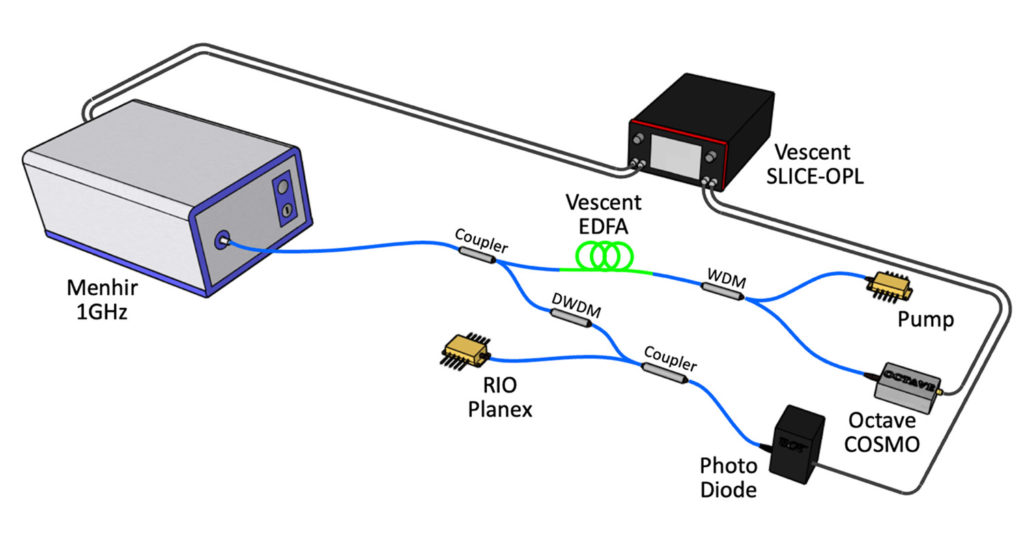
Figure 1. Breadboard demonstration of a high repetition rate optical frequency comb based on a Menhir 1 GHz mode-locked oscillator, an Octave Photonics COSMO fceo detector, and Vescent SLICE Offset Phase Locking (OPL) electronics. A dispersion managed single-pumped Vescent erbium doped fiber amplifier (EDFA) was used to amplify and compress the pulses from the Menhir oscillator together with fceo detection in an Octave COSMO. A narrow linewidth RIO Planex laser was used for fopt detection after filtering the Menhir oscillator light with a dense wavelength division multiplexer (DWDM), combining the two sources in a fiber splitter, and directing these signals onto a fast photodetector. Both fopt and fceo were then sent to high-speed offset phase locking electronics (Vescent SLICE-OPL) where laser heterodyne signals can be phase-locked to an RF oscillator. In-loop phase noise was measured with an external phase noise analyzer (Microchip 53100A, not shown).
Vescent Photonics has prototyped a ruggedized, compact, fully stabilized breadboard optical frequency comb based on commercially available components (Figure 1). The subcomponents include a commercial 1 GHz oscillator, a single core-pumped fiber amplifier, an fceo detector based on a nanophotonics waveguide, a narrow linewidth laser, and high-bandwidth phase locking electronics. The optical system is comprised of all-polarization maintaining (PM) fiber components to simplify assembly and to allow for reliable long-term operation. The oscillator is a commercial 1 GHz mode-locked laser (Menhir-1550) known for its exceptionally low phase noise and excellent longevity. An EDFA and dispersion management are used to generate pulses with 180 fs duration (FWHM) and 140 pJ pulse energy which are then fed into an offset frequency detector (Octave Photonics COSMO) for generation of fceo. To stabilize an optical tooth of the comb, we generate a heterodyne beat signal (fopt) with a narrow linewidth cw laser (RIO Planex) after filtering the comb light with a telecom DWDM filter. Both signals show a signal-to-noise ratio (SNR) of >50dB (100kHz RBW) which is more than sufficient for reliable long-term operation. The two signals are locked to an RF reference using Vescent SLICE-OPL locking electronics by feeding back to the pump current of the oscillator and intra-cavity PZTs, respectively.
2. Results
The free-running comb RF beat signals are shown in Figure 2a) and b). We lock fceo with a residual integrated phase noise of 265 mrad (Figure 2c) while the stabilized fopt shows a residual phase noise of 273 mrad (Figure 2d, both integrated from 1 kHz to 1 MHz). It should be noted that the noise in the optical tooth lock is limited by the noise of the cw laser used to generate fopt. Better performance can be achieved when using, for example, a cavity-stabilized cw laser [6].
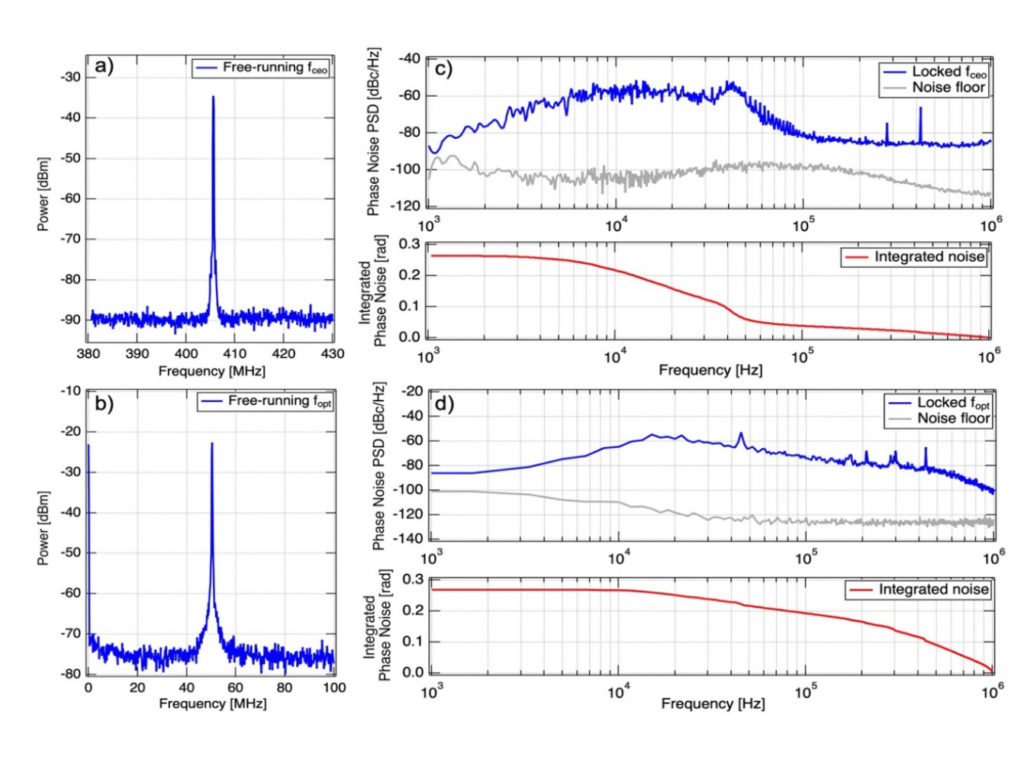
Figure 2: a) and b): RF spectrum of the free-running fceo and fopt beat notes (100 kHz RBW). c) and d): Phase noise power spectral density of the locked fceo and fopt with their integrated phase noise below. fceo and fopt are stabilized with a residual integrated phase noise of 265 mrad and 273mrad (from 1 kHz to 1 MHz), respectively.
Finally, preliminary frequency instability (Allan deviation) measurements have been calculated from the
in-loop phase noise PSDs and extrapolated to longer time scales (Figure 3). Vescent is actively collecting additional data on this system to measure the frequency instability at timescales greater than 1 s. Previous measurements on similar systems indicate, however, that the in-loop phase noise is an excellent indicator of the Allan deviation performance at longer time scales. This level of optical stability shows that these high repetition rate optical frequency combs are suitable for high performance applications such as optical atomic clocks, dual comb spectroscopy, and low phase noise microwave generation.
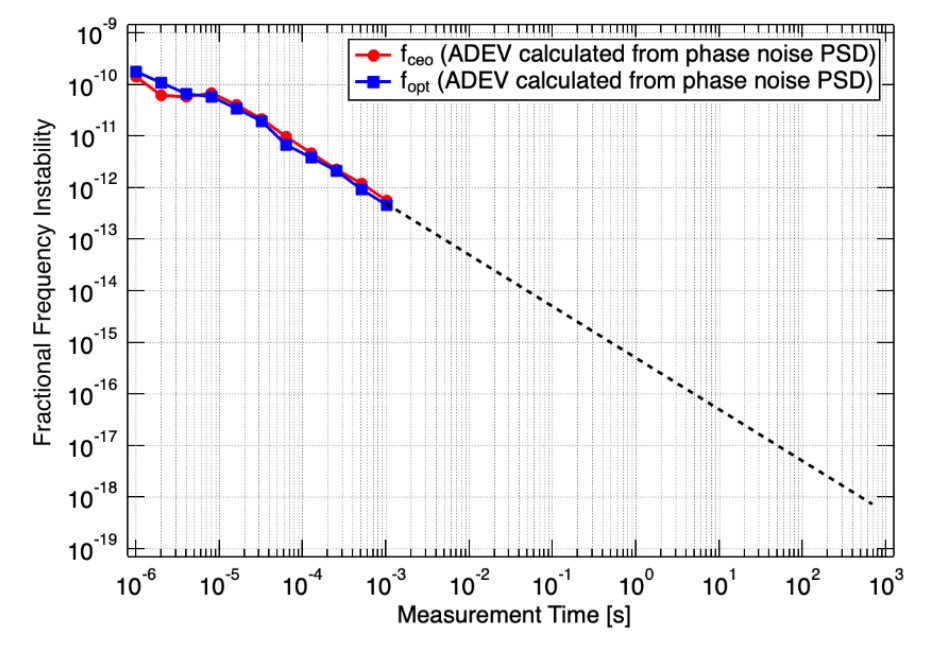
Figure 3: Initial frequency instability measurements of fceo (red circles) and fopt (blue squares) as calculated from the in-loop phase noise PSD. Data collection is on-going to provide in-loop stabilities at timescales >> 1 s.
3. References
- D.I. Herman et al., “Precise multispecies agricultural gas flux determined using broadband open-pathdual-comb spectroscopy,” Science Advances, vol. 7, no. 14, 2021.
- G.B. Rieker et al., “Frequency-comb-based remote sensing of greenhouse gases over kilometer air paths,” Optica, vol. 1, no. 290-298, 2014.
- Tze-An Liu et al., “Sub-micron absolute distance measurements in sub-millisecond times with dual
free-running femtosecond Er fiber-lasers,” Optics Express, vol. 19, no. 19, pp. 18501-18509, 2011. - A.J. Metcalf et al., “Stellar spectroscopy in the near-infrared with a laser frequency comb,” Optica,
vol. 6, pp. 233-239, 2019. - “Menhir Photonics,” [Online]. Available: https://menhir-photonics.com.
- D.M.B. Lesko et al., “Fully phase-stabilized 1 GHz turnkey frequency comb at 1.56 μm,” OSA Cont.,
vol. 3, 2020.
Download |
Search
Popular posts
Popular tags